COAST IMPACT - Individual and Population Effects of Multiple Stressors
COAST IMPACT
COAST IMPACT is a research project funded by the Norwegian Research Council that began in August 2020. The main aim of the project is to investigate the influence of pollution and infectious diseases on shorebirds that migrate along the East Asian–Australasian Flyway (EAAF).
The EAAF is one of the nine major flyways around the world extending all the way from Arctic Russia and North America (where migratory shorebirds spend the breeding season), southwards to East Asia and Australia (where migratory shorebirds spend the non-breeding season). Every year, millions of shorebirds migrate along this flyway.
The coastal wetlands of East Asia are critical stopover and staging sites; locations en route where the birds can rest and refuel, respectively. Unfortunately, the expeditious economic development across East Asia has resulted in significant degradation, pollution and losses of these important habitats. This rapid decline in habitat quantity and quality further increases the myriad of stressors that these birds are exposed to during this high energy-demanding migratory period.
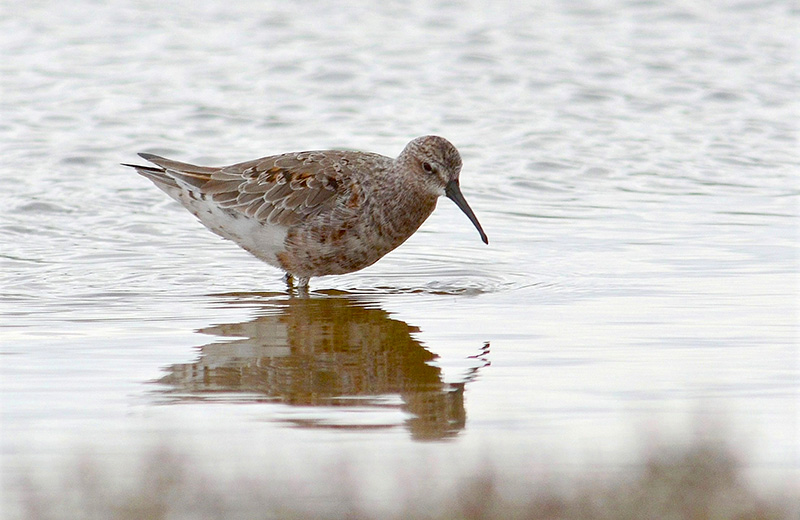
Data shows that populations of shorebirds that migrate along the EAAF are drastically decreasing1. The cause of these declines has been largely attributed to anthropogenic activities. These activities have resulted in, among others, decreased habitat size and food availability and an increased exposure to mixtures of chemicals and various pathogens. Pollution exposure modulates the immune system and could therefore lead to a higher prevalence of infectious diseases. These diseases could not only decrease shorebird survival rates but may also increasingly spread to humans (e.g. avian influenza viruses). However, these processes are poorly understood.
Recently, microRNAs have been identified as key regulators of gene expression and important determinants of disease outcomes. MicroRNAs are a class of small noncoding RNAs that bind specific mRNA transcripts and prevent their translation into proteins. MicroRNAs are critical in various important biological processes and alternations in their expression have been found after exposure to various pollutants2. Recent in vitro studies suggest that microRNAs may be a mechanistic link in our understanding of pollutant-induced immunomodulation3,4. The presence and stability of microRNAs in blood makes them potentially interesting biomarkers of pollution-induced disease outcomes.
There is a great need for a better understanding of the specific factors that affect migratory shorebird survival. COAST IMPACT will therefore investigate:
- Pollutant exposure during migration
- Which important coastal wetlands in East Asia present the most polluted hot spots
- How the diversity and biomass of food sources is affected by the pollution at those sites
- Which pollutants are found in the highest concentrations in the shorebirds upon arrival in Australia
- How tracking data can be used to relate pollutant concentrations in shorebirds to pollutant exposure during migration
- Effects of pollutant exposure during migration on survival and infectious disease susceptibility
- If the level of pollution at the stopover/staging sites predicts survival
- If biodiversity of food sources at the stopover/staging sites predicts survival
- If birds with high pollutant concentrations are more likely to be infected with avian influenza and other pathogens
- If microRNA profiles can be used as novel biomarkers for exposure to pollution and pathogens
The outcome of this project will give insight into the mechanisms that drive population changes in migrating shorebirds. Elucidating the role of the various threats in the actual declines will allow more targeted conservation efforts.
Videos
PhD candidate Anne-Fleur Brand studies the combined influence of pollution and infectious diseases on EAAF shorebirds.
Postdoc Dr. Junjie Zhang studies the detection and analysis of pollutants in samples from EAAF shellfish, shorebirds and coastal sediments.
Approach
As part of a long-term ongoing monitoring programme in Australia, coordinated by project partner Prof. Marcel Klaassen (Deakin University, Australia), 7000+ samples (red blood cells, serum, cloacal/pharyngeal swabs) from migratory shorebirds are available. From this extensive archive, a subset of red blood cells (n = 110) was analysed for per- and polyfluoroalkyl substances8 with funding from the Australian Research Council (ARC). Within the framework of the same ARC project, metals were recently analysed in red blood cells.
COAST IMPACT will expand this dataset through analysis of shorebird blood as well as sediment and benthos samples for a wider range of contaminants, including PCBs, flame retardants, organochlorine pesticides, parabens, phthalates, benzophenone UV-filters and bisphenols. These samples will be collected from Australia as well as from the Yellow Sea region and Arctic Russia.
Prof. Veerle Jaspers (Department of Biology) in the project coordinator and Assoc. Prof. Courtney Waugh (Nord University) is the co-PI. PhD candidate Anne-Fleur Brand (Department of Biology) and postdoc Dr Junjie Zhang (Department of Chemistry) are hired on the project.
Close collaborations with Assoc. Prof. Alexandros Asimakopoulos (NTNU Department of Chemistry), Prof. Adrian Covaci (University of Antwerp, Belgium) and Dr Dorte Herzke (Norwegian Institute for Air Research, Norway) enable us to prepare and analyse samples for a wide range of pollutants. Other project partners include Prof. Lei Wang (Nankai University, China) and Hebo Peng (University of Groningen, Netherlands & Beijing Forest University, China).
References
- Studds, C. E., Kendall, B. E., Murray, N. J., Wilson, H. B., Rogers, D. I., Clemens, R. S., Gosbell, K., Hassell, C. J., Jessop, R. & Melville, D. S. 2017. Rapid population decline in migratory shorebirds relying on Yellow Sea tidal mudflats as stopover sites. Nature communications, 8, 1-7.
- Krauskopf, J., De Kok, T. M., Hebels, D. G., Bergdahl, I. A., Johansson, A., Spaeth, F., Kiviranta, H., Rantakokko, P., Kyrtopoulos, S. A. & Kleinjans, J. C. 2017. MicroRNA profile for health risk assessment: environmental exposure to persistent organic pollutants strongly affects the human blood microRNA machinery. Scientific reports, 7, 1-9.
- Badry, A., Jaspers, V. L. & Waugh, C. A. 2020. Environmental pollutants modulate RNA and DNA virus-activated miRNA-155 expression and innate immune system responses: Insights into new immunomodulative mechanisms. Journal of Immunotoxicology, 17, 86-93.
- Waugh, C. A., Arukwe, A. & Jaspers, V. L. 2018. Deregulation of microRNA‐155 and its transcription factor NF‐kB by polychlorinated biphenyls during viral infections. Apmis, 126, 234-240.
- Skaalvik, T.G. 2020. Per- and polyfluoroalkyl substances in shorebirds of Australia. (Master’s thesis).
References SETAC Copenhagen 2022 poster ID 8108
- Hoye, B.J., Donato, C.M., Lisovski, S., Deng, Y.M., Warner, S., Hurt, A.C., Klaassen, M. and Vijaykrishna, D., 2021. Reassortment and persistence of influenza A viruses from diverse geographic origins within Australian wild birds: evidence from a small, isolated population of Ruddy turnstones. Journal of virology, 95(9), pp.e02193-20.
- Wille, M., Lisovski, S., Risely, A., Ferenczi, M., Roshier, D., Wong, F.Y., Breed, A.C., Klaassen, M. and Hurt, A.C., 2019. Serologic evidence of exposure to highly pathogenic avian influenza H5 viruses in migratory shorebirds, Australia. Emerging infectious diseases, 25(10), p.1903.
- Victorian Wader Study Group, 2022. Ruddy turnstone, geolocator studies.
- Sun, J., Letcher, R.J., Waugh, C.A., Jaspers, V.L., Covaci, A. and Fernie, K.J., 2021. Influence of perfluoroalkyl acids and other parameters on circulating thyroid hormones and immune-related microRNA expression in free-ranging nestling peregrine falcons. Science of The Total Environment, 770, p.145346.
- Waugh, C.A., Arukwe, A. and Jaspers, V.L., 2018. Deregulation of microRNA‐155 and its transcription factor NF‐kB by polychlorinated biphenyls during viral infections. Apmis, 126(3), pp.234-240.
- Jia, X., Li, X., Shen, Y., Miao, J., Liu, H., Li, G. and Wang, Z., 2016. MiR‐16 regulates mouse peritoneal macrophage polarization and affects T‐cell activation. Journal of cellular and molecular medicine, 20(10), pp.1898-1907.
- Rosenberger, C.M., Podyminogin, R.L., Navarro, G., Zhao, G.W., Askovich, P.S., Weiss, M.J. and Aderem, A., 2012. miR-451 regulates dendritic cell cytokine responses to influenza infection. The Journal of Immunology, 189(12), pp.5965-5975.
- Eslamloo, K., Inkpen, S.M., Rise, M.L. and Andreassen, R., 2018. Discovery of microRNAs associated with the antiviral immune response of Atlantic cod macrophages. Molecular immunology, 93, pp.152-161.
- Ghadiri, N., Emamnia, N., Ganjalikhani-Hakemi, M., Ghaedi, K., Etemadifar, M., Salehi, M., Shirzad, H. and Nasr-Esfahani, M.H., 2018. Analysis of the expression of mir-34a, mir-199a, mir-30c and mir-19a in peripheral blood CD4+ T lymphocytes of relapsing-remitting multiple sclerosis patients. Gene, 659, pp.109-117.
- Roy, S., Benz, F., Vargas Cardenas, D., Vucur, M., Gautheron, J., Schneider, A., Hellerbrand, C., Pottier, N., Alder, J., Tacke, F. and Trautwein, C., 2015. miR‐30c and miR‐193 are a part of the TGF‐β‐dependent regulatory network controlling extracellular matrix genes in liver fibrosis. Journal of digestive diseases, 16(9), pp.513-524.
- Yin, X., Ge, J., Ge, X., Gao, J., Su, X., Wang, X., Zhang, Q. and Wang, Z., 2021. MiR‐363‐5p modulates regulatory T cells through STAT4‐HSPB1‐Notch1 axis and is associated with the immunological abnormality in Graves' disease. Journal of cellular and molecular medicine, 25(19), pp.9364-9377.
- Li, R.D., Shen, C.H., Tao, Y.F., Zhang, X.F., Zhang, Q.B., Ma, Z.Y. and Wang, Z.X., 2018. MicroRNA-144 suppresses the expression of cytokines through targeting RANKL in the matured immune cells. Cytokine, 108, pp.197-204.
- Yu, D.I., Tan, A.H.M., Hu, X., Athanasopoulos, V., Simpson, N., Silva, D.G., Hutloff, A., Giles, K.M., Leedman, P.J., Lam, K.P. and Goodnow, C.C., 2007. Roquin represses autoimmunity by limiting inducible T-cell co-stimulator messenger RNA. Nature, 450(7167), pp.299-303.
- Sun, Q., Wang, S., Chen, J., Cai, H., Huang, W., Zhang, Y., Wang, L. and Xing, Y., 2019. MicroRNA‐190 alleviates neuronal damage and inhibits neuroinflammation via Nlrp3 in MPTP‐induced Parkinson's disease mouse model. Journal of cellular physiology, 234(12), pp.23379-23387.
- Yu, Y., Zhang, D., Huang, H., Li, J., Zhang, M., Wan, Y., Gao, J. and Huang, C., 2014. NF-κB1 p50 promotes p53 protein translation through miR-190 downregulation of PHLPP1. Oncogene, 33(8), pp.996-1005.
- Xu, Z., Xiao, S.B., Xu, P., Xie, Q., Cao, L., Wang, D., Luo, R., Zhong, Y., Chen, H.C. and Fang, L.R., 2011. miR-365, a novel negative regulator of interleukin-6 gene expression, is cooperatively regulated by Sp1 and NF-κB. Journal of Biological Chemistry, 286(24), pp.21401-21412.
- Guan, T., Dominguez, C.X., Amezquita, R.A., Laidlaw, B.J., Cheng, J., Henao-Mejia, J., Williams, A., Flavell, R.A., Lu, J. and Kaech, S.M., 2018. ZEB1, ZEB2, and the miR-200 family form a counterregulatory network to regulate CD8+ T cell fates. Journal of Experimental Medicine, 215(4), pp.1153-1168.
- Wu, W., He, C., Liu, C., Cao, A.T., Xue, X., Evans-Marin, H.L., Sun, M., Fang, L., Yao, S., Pinchuk, I.V. and Powell, D.W., 2015. miR-10a inhibits dendritic cell activation and Th1/Th17 cell immune responses in IBD. Gut, 64(11), pp.1755-1764.
- Jiang, S., 2018. Recent findings regarding let-7 in immunity. Cancer letters, 434, pp.130-131.
- Lu, R.H., Jia, S.Z., Yang, F., Qin, C.B., Zhang, Y.R., Meng, X.L., Yan, X., Feng, J.C. and Nie, G.X., 2020. The function of miR-122 in the lipid metabolism and immunity of grass carp (Ctenopharyngodon idellus). Aquaculture Reports, 17, p.100401.
- Xiao, C., Srinivasan, L., Calado, D.P., Patterson, H.C., Zhang, B., Wang, J., Henderson, J.M., Kutok, J.L. and Rajewsky, K., 2008. Lymphoproliferative disease and autoimmunity in mice with increased miR-17-92 expression in lymphocytes. Nature immunology, 9(4), pp.405-414.
- Hsin, J.P., Lu, Y., Loeb, G.B., Leslie, C.S. and Rudensky, A.Y., 2018. The effect of cellular context on miR-155-mediated gene regulation in four major immune cell types. Nature immunology, 19(10), pp.1137-1145.
- Liu, C., Wang, J. and Zhang, X., 2014. The involvement of MiR-1-clathrin pathway in the regulation of phagocytosis. PLoS One, 9(6), p.e98747.
- Pareek, S., Sanchenkova, X., Sakaguchi, T., Murakami, M., Okumura, R., Kayama, H., Kawauchi, S., Motooka, D., Nakamura, S., Okuzaki, D. and Kishimoto, T., Epithelial miR‐215 negatively modulates Th17‐dominant inflammation by inhibiting CXCL12 production in the small intestine. Genes to Cells.
- Ross, T. et al. Manuscript in preparation.
- Friedländer, M.R., Mackowiak, S.D., Li, N., Chen, W. and Rajewsky, N., 2012. miRDeep2 accurately identifies known and hundreds of novel microRNA genes in seven animal clades. Nucleic acids research, 40(1), pp.37-52.
- Moraga, C., Sanchez, E., Ferrarini, M.G., Gutierrez, R.A., Vidal, E.A. and Sagot, M.F., 2020. BrumiR: A toolkit for de novo discovery of microRNAs from sRNA-seq data. bioRxiv.
- Love, M.I., Huber, W. and Anders, S., 2014. Moderated estimation of fold change and dispersion for RNA-seq data with DESeq2. Genome biology, 15(12), pp.1-21.