Ola H. Ramstad
Neural disorder at the edge of chaos
Ola Huse Ramstad
Research Group: Translational Neuroscience group. NTNU Cyborg project.
Department of Neuromedicine and Movement Science, Faculty of Medicine and Health Sciences
When a nervous system is impaired, we see this as symptoms in the behavior or condition of those unfortunate enough to be affected, such as tremors in those with Parkinson’s or seizures in those with epilepsy.
Yet for many such disorders there is no apparent physical cause for the symptoms, no clear culprit to aim our medicine at. While some forms of epilepsy stem from a clearly diagnosed tumor, other forms arise from subtle shifts in the communication between neurons, like telephone signals just slightly out of synch with each other.
If we can understand the normal communication between neurons, we can detect when these subtle shifts occur, and predict when the communication moves from function to dysfunction. We are not there yet of course, there is still infinitely much we don’t understand about how our neurons communicate, and further still, how this communication gives rise to the behaviors we see.
But by describing changes in network communication, how the signal travels along the telephone wire you could say, we could detect when this communication was changing and how the neurons responded to an injury. This focus on network communication also provides us with insight on how one might start to mitigate such a disorder, that is, how do we bring the neurons back to their former function. So, when a spinal cord is injured, we might instead focus our efforts at restoring the connections, or wires, that provide the most relief and greatest function to the injured, instead of trying to restore all the connections at once.
So how did we study this in the lab? In practical terms, much our work was aimed at models of neurons, starting by creating neurons from stem cells, then describing the communication between these neurons using mathematical models. By working with the NTNU Nanolab we were fortunate enough to create microstructures to place our neurons within and better test and control their injuries. Along with providing control, these structures also contained microelectrodes which gave us a window into the electrical communication of our neurons. Then when placing spinal cord neurons inside these structures we could see how the neurons responded (or didn’t respond) when we created a simulated spinal cord injury.
Alongside this we worked with the AI lab at OsloMet to utilize a concept in chaos theory, termed criticality, to describe the interaction between our neurons. Criticality within neural systems is thought to be a sign of efficient communication, and the change within our neurons from a critical state to a non-critical state of communication may be the sort of subtle shift that we seek. Ultimately there are no clear answers, but our tools provide hints of when and why networks communicate effectively, when they don’t, and how to turn one into the other. By using models and tools, we may in the future find the subtle shift or missing telephone wire that means the difference between function and dysfunction in our neurons.
Main supervisors: Lars Jacob Stovner & Axel Sandvig
Project period: 2017-2020
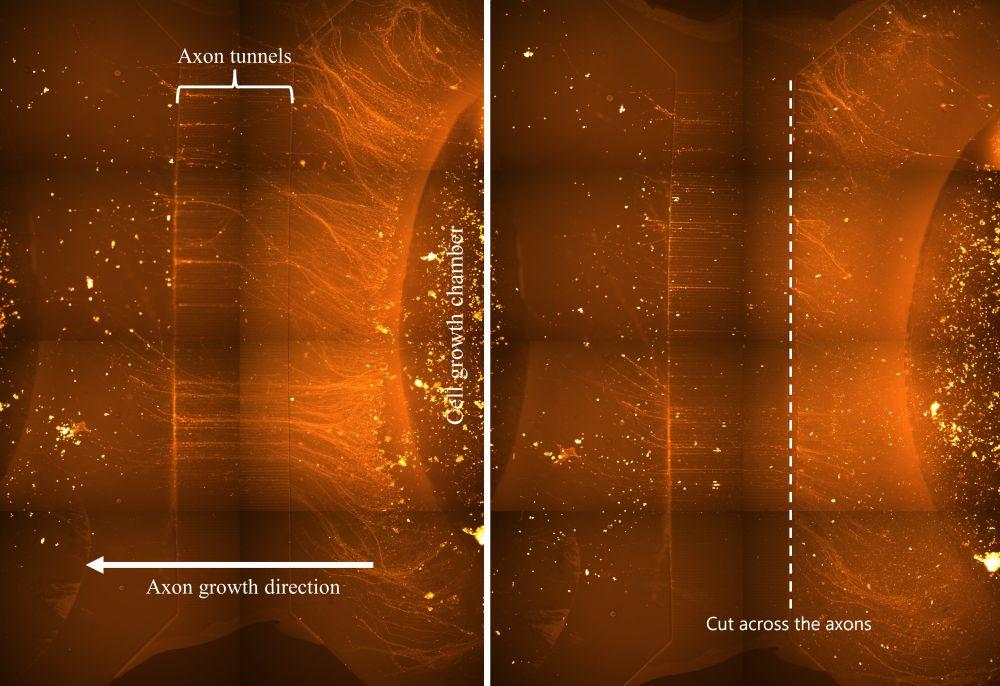