Discovering the Grid Cells, the Kavli Institute
Discovering grid cells
This is the story of how the Mosers discovered grid cells, as told by themselves.
We both grew up on remote islands off the west coast of Norway, a couple of hundred miles north of Bergen. This was not exactly a center of academic ferment or intellectual competition. Still, our scientific interests were nurtured by parents who did not have the opportunity to get an education themselves. We went to the same high school, but didn't really get to know each other until we met again at the University of Oslo in the 1980s.
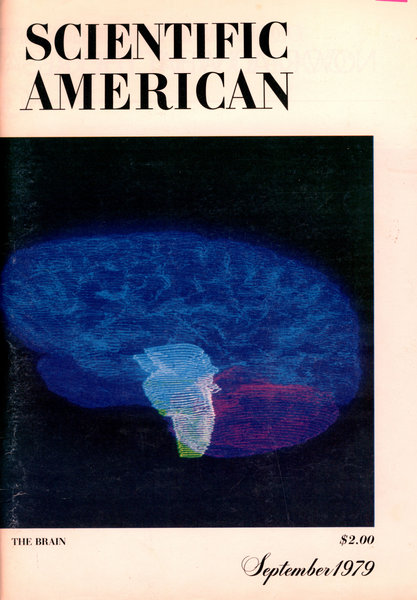
Scientific American "Brain Issue"
Scientific American - the 1979 brain issue
With no clear career plan, and with different scientific backgrounds, we met in an undergraduate course in psychology. Psychology both reinforced and kindled our fascination with the brain and we jointly decided to find out how we could learn about the neural basis of behavior. There was no neuroscience curriculum at the time, but Carl Erik Grenness, who taught an undergraduate course on behavior analysis, alerted the two of us to the pioneering work on brain-behaviour relationships that was taking place at this time. He also gave us a copy of a special issue about the brain published by Scientific American in 1979. During our wandering in wilderness, this was like manna from heaven. The issue conveyed the enthusiasm in the field and strongly attracted us to this evolving scientific discipline. Among the advances that were reviewed were Kandel's demonstration of synaptic mechanisms of memory in Aplysia californica, and Hubel and Wiesel's characterization of the mechanism for feature analysis in the visual cortex.
Meeting Per Andersen
Grenness also sent us to Terje Sagvolden, the only psychologist at the university with research projects in neuroscience at that time. We worked on neurochemical mechanisms of attention deficit disorder for two years, in parallel with studies in psychology, and were taught the basics of animal behavior and experimental design. This triggered our interest in animal learning. That brought us to visit Per Andersen, the grand neurophysiologist of Norway. He was a great physiologist and we were interested in the intersection of behavior and physiology. We sat there for hours, trying to persuade him to take us on as graduate students. He really couldn't get us out of his office, and we wouldn't take no for an answer. In the end, he yielded to our combination of furious curiosity and unwavering determination and took us on.
Bomb shelter lab
Per Andersen became our PhD supervisor and introduced us to the mysteries of the brain. We learned to focus on basic questions with broad implications. Through Per, we came in touch with Richard Morris at the University of Edinburgh and John O'Keefe at University College London. Richard and John were the best mentors we could have had. They guided us into the mysteries at the intersection of behavior and neuroscience. During our PhD studies, we visited Richard several times to participate in work on the functions of the hippocampus and the role of hippocampal long-term potentiation in memory formation. After our PhD defense late in 1995, we spent a few very rewarding months with John to learn place-cell recording in the hippocampus. This was probably the most intense learning experience in our lives. But then, in 1996, we were almost ambushed with a job offering in Trondheim. We could not move there if only one of us got a job, so we negotiated two jobs as well as equipment to start up a new lab. We literally started our lab in a "bomb shelter" in a basement at the university. Our few months of postdoctoral experience had been brief, but with a decent start-up package we now had the opportunity to combine what we had learned about animal behavior and neurophysiology, fulfilling our dream from the early 1980s. We began recording the activity of individual cells in the hippocampus, with electrodes implanted in the brains of rats as they roamed a square black box.
Our start-up in Trondheim was tough but enjoyable. There were no animal housing facilities, no workshops and no technicians. We did all the work on our own; we cleaned rat cages, changed bedding, sliced brains and repaired cables. Starting from scratch gave us the opportunity to shape the lab exactly as we wanted it.
- Where do the hippocampal signals come from?
As we started up, we got a grant from the European Commission to coordinate a consortium of seven groups that collectively aimed to perform one of the first integrated neural network studies of hippocampal memory. This was virgin territory in the late 1990s. One of the aims was to determine how the position code of the hippocampus is computed. It had been known since 1971 that the hippocampus has "place cells," cells that fire if and only if an animal is in a certain place. But it was unclear whether those place signals originated in the hippocampus itself or came from the outside. To address this question, we made intrahippocampal lesions that disconnected the output stage of the circuit – CA1 – from the earlier stages. To our surprise, this did not abolish place coding in CA1. Surprised, we had to grapple with the idea that the spatial signal might originate from somewhere else, most likely the surrounding cortex, through connections that went around the intrahippocampal circuit. The most likely candidate was the entorhinal cortex, a cortical region with major direct connections to the CA1 area of the hippocampus.
We started recording in this region, and got invaluable help from Menno Witter, a neuroanatomist who was then located at the Free University of Amsterdam, but later moved to become a part of the Kavli Institute in Trondheim. Witter had by that time worked out much of the connectivity between the entorhinal cortex and hippocampus and helped us in the delicate task of guiding electrodes to the right spot. By 2002, the research group had grown and we now had an outstanding team of students working side by side with us in the lab and on the computer.
The long road to realization
Sometimes scientific discoveries are portrayed as "Eureka" moments, where the researcher suddenly understands the significance of what he or she has found. In our case, it didn't quite work that way: We didn't immediately realize that the cells we recorded from were grid cells. At first we noticed that many entorhinal cells spiked every time a rat went to a particular spot, like the place cells in the hippocampus. However, each cell had multiple firing locations and those firing locations formed a peculiarly regular pattern – a hexagonal grid – much like the arrangement of marbles in a Chinese checker board. Every cell did it this way, with actual firing locations differing between cells. The cells were organized topographically in the sense that the size of and distance between grid fields increased from dorsal to ventral. Moreover, cells maintained firing relationships from one environment to the next, suggesting that we were on track of a universal type of spatial map – a map whose activity pattern in many ways disregarded the fine details of the environment. With their strict regularity, the cells had the metrics of the spatial map that had not been found in the hippocampus.
Grid cells and beyond
These discoveries were published in a series of papers that began in 2004, only two years after we published the hippocampal disconnection study. The grid pattern itself was published in 2005. Since then we have continued to explore how grid cells operate, how they are generated, and how they interact with other spatial cell types. There is still a lot to find out. Grid cells have helped us better understand the neural representation of space, but they also provide a window into some of the innermost workings of the brain. Perhaps the most fascinating thing is that the hexagonal pattern is generated by the cortex itself. There is no grid pattern in the outside world – this is made by the brain alone. Because the pattern is so reliable and so regular, it may put us on the track of understanding the fundamental computations of the cortex.
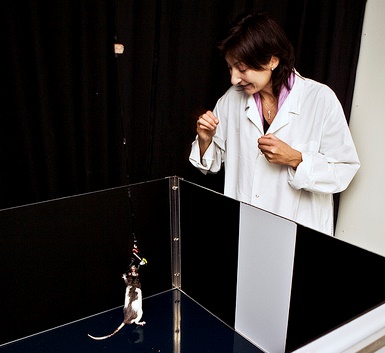
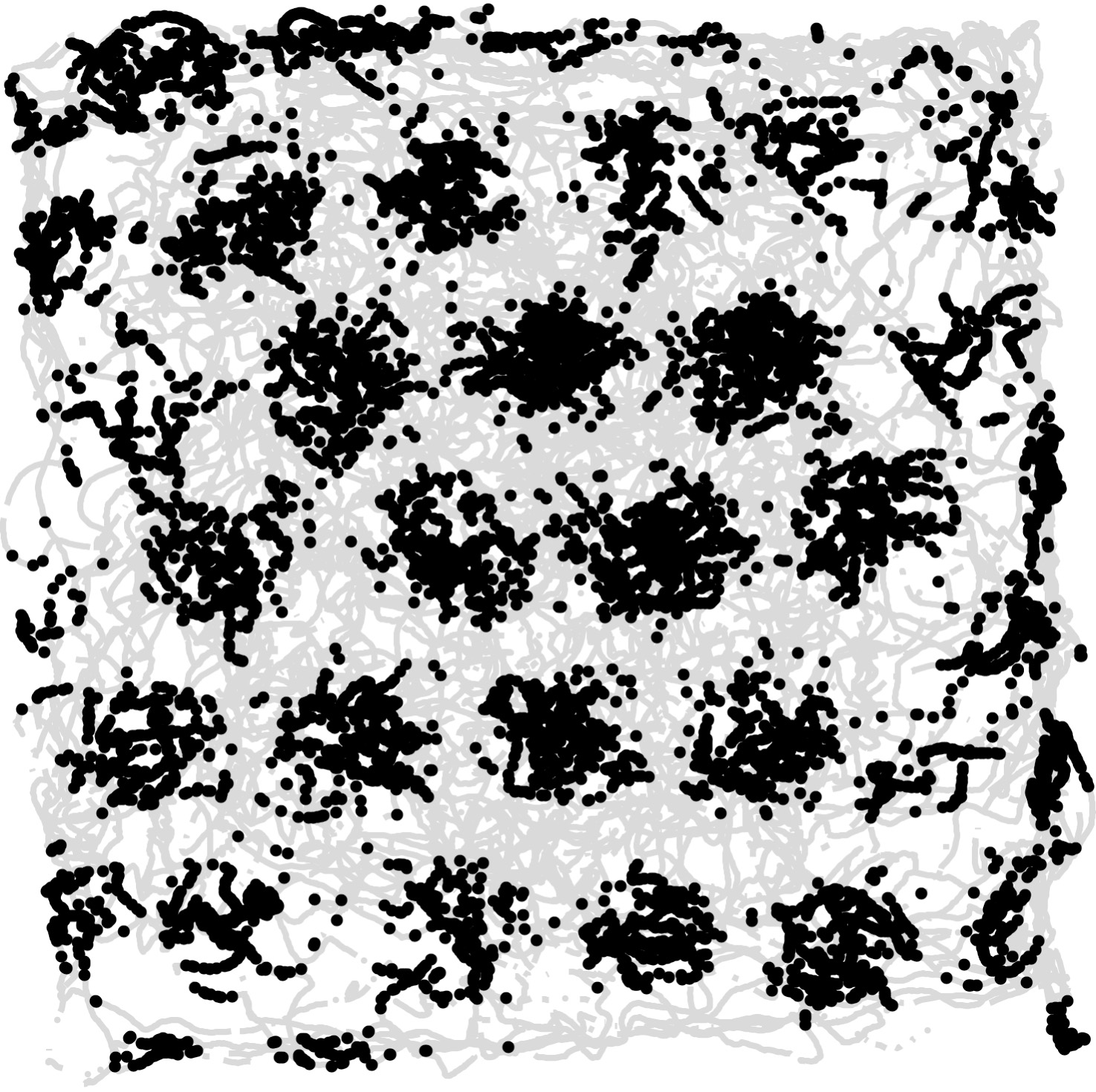
Selected grid cell articles
Brun, V.H., Otnæss, M.K., Molden, S., Steffenach, H.-A., Witter, M.P., Moser, M.-B., Moser, E.I. (2002). Place cells and place recognition maintained by direct entorhinal-hippocampal circuitry. Science, 296, 2243-2246.
Fyhn, M., Molden, S., Witter, M.P., Moser, E.I. and Moser, M.-B. (2004). Spatial representation in the entorhinal cortex. Science, 305, 1258-1264.
Leutgeb, S., Leutgeb, J.K., Treves, A., Moser, M.-B. and Moser, E.I. (2004). Distinct ensemble codes in hippocampal areas CA3 and CA1. Science 305, 1295-1298.
Leutgeb, S., Leutgeb, J.K., Barnes, C.A., Moser, E.I., McNaughton, B.L., and Moser, M.-B (2005). Independent codes for spatial and episodic memory in the hippocampal neuronal ensembles. Science, 309, 619-623.
Hafting, T., Fyhn, M., Molden, S., Moser, M.-B., and Moser, E.I. (2005). Microstructure of a spatial map in the entorhinal cortex. Nature, 436, 801-806.
Sargolini, F., Fyhn, M., Hafting, T., McNaughton, B.L., Witter, M.P., Moser, M.-B., and Moser, E.I. (2006). Conjunctive representation of position, direction and velocity in entorhinal cortex. Science, 312, 754-758.
Fyhn, M., Hafting, T., Treves, A., Moser, M.-B. and Moser, E.I. (2007). Hippocampal remapping and grid realignment in entorhinal cortex. Nature, 446, 190-194.
Hafting, T., Fyhn, M., Bonnevie, T., Moser, M.-B. and Moser, E.I. (2008). Hippocampus-independent phase precession in entorhinal grid cells. Nature 453, 1248-1252.
Kjelstrup, K.B., Solstad, T., Brun, V.H., Hafting, T., Leutgeb, S., Witter, M.P., Moser, E.I. and Moser, M.-B. (2008). Finite scales of spatial representation in the hippocampus. Science 321, 140-143.
Solstad, T., Boccara, C.N., Kropff, E., Moser, M.-B. and Moser, E.I. (2008). Representation of geometric borders in the entorhinal cortex. Science, 322, 1865-1868.
Langston RF, Ainge J, Cowey JJ, Canto CB, Bjerknes TL, Witter MP, Moser EI, Moser M-B (2010). Development of the spatial representation system in the rat. Science, 328, 1576-1580.
Giocomo LM, Hussaini SA, Zheng F, Kandel ER, Moser M-B and Moser EI (2011). Grid cells use HCN1 channels for spatial scaling. Cell, 147, 1159-1170.
Stensola H, Stensola T, Solstad T, Frøland K, Moser M-B and Moser EI (2012). The entorhinal grid map is discretized. Nature, 492, 72-78.
Couey JJ, Witoelar A, Zhang S-J, Zheng K, Ye J, Dunn B, Czajkowski R, Moser M-B, Moser EI, Roudi Y, Witter MP (2013). Recurrent inhibitory connectivity between entorhinal layer II stellate cells is sufficient to generate grid-cell patterns. Nature Neurosci., 16, 318-324.
Bonnevie T, Dunn B, Fyhn M, Hafting T, Derdikman D, Kubie JL, Roudi Y, Moser EI and Moser M-B (2013). Grid cells require excitatory drive from the hippocampus.Nature Neurosci., 16, 309-317.
Zhang S-J, Ye J, Miao C, Tsao A, Cerniauskas I, Ledergerber D, Moser M-B and Moser EI (2013). Optogenetic dissection of entorhinal-hippocampal functional connectivity. Science, 340, 1232627.